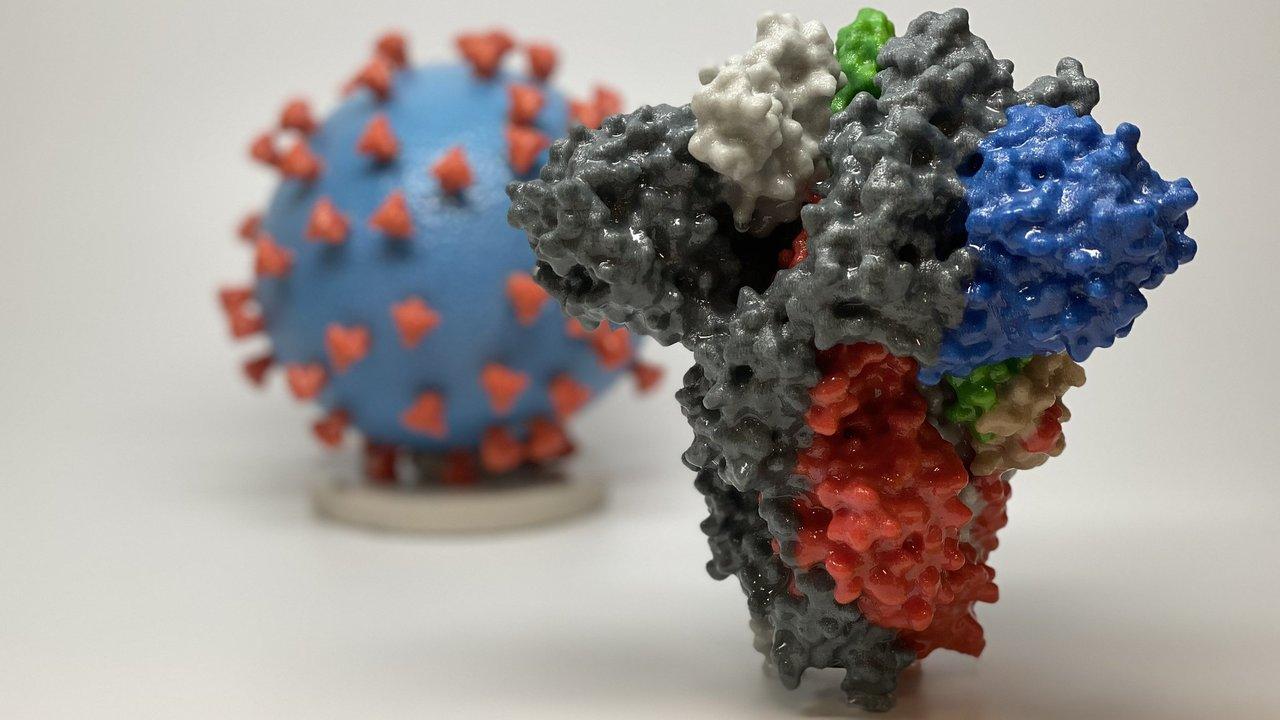
Making proteins for COVID-19 antibody tests
Three chemical engineering research groups are teaming up to find new ways to produce COVID-19 proteins for serological tests for antibodies. With a new grant from the UC Davis Office of Research COVID-19 Research Accelerator Funding Track (CRAFT), assistant professor Priya Shah and professors Karen McDonald and Roland Faller are combining their expertise to quickly produce high-quality viral proteins using mammalian cells and plants.
“This is a great example of where microbiology and virology meet chemical engineering,” said Shah. “I think it really nicely brings together multiple labs in the chemical engineering department to make progress on an important problem.”
To reach herd immunity—an important step toward safely re-opening the country—Johns Hopkins University estimates that 70% of the population needs to have COVID-19 antibodies, making regular testing necessary for millions of people. This means producing millions, if not billions, of serological tests, which requires an even larger number of COVID-19 proteins.
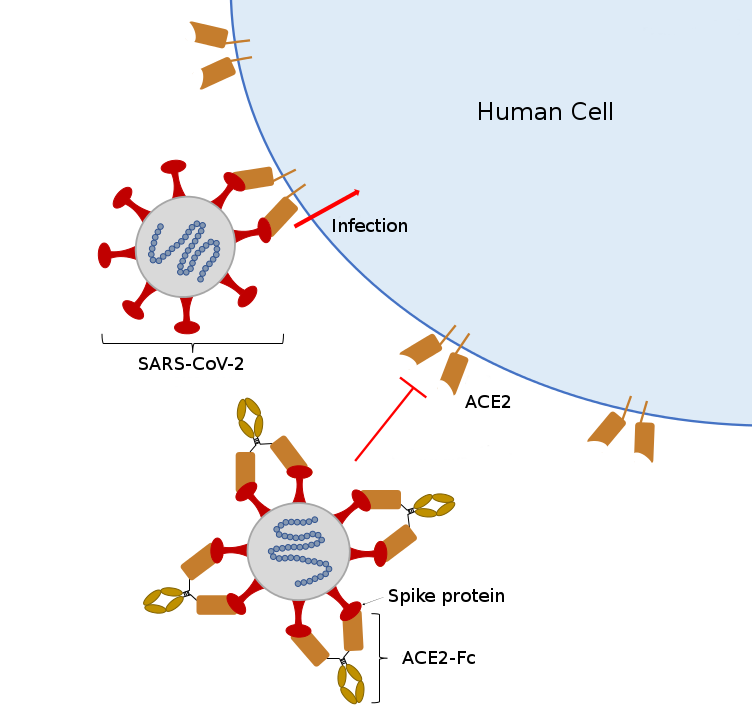
“All of these tests have lots of different reagents in them and if you’re limited in any one, you’re limited in the testing you can do,” said McDonald. “So we’re working on research to provide one very important reagent, which is the functional protein spike that is going to bind to the antibodies.”
Spike proteins and sugars
Spike proteins are the little spikes that surround a coronavirus and make it look like a sun. These proteins are the virus’ tool for binding with cells. During an infection, antibodies go after these proteins to stop them from binding to other cells. If a sample has these antibodies, they will identify and bind to protein and the serological test will come back positive.
Because spike proteins are glycosylated, or modified by sugars, they are difficult to produce in bacteria, but ideal for mammalian or plant cells. This is where Shah and McDonald come in. McDonald and collaborator Somen Nandi’s lab uses plants to produce proteins for human drugs, and now, for diagnostics. By hijacking plant virus replication machinery, they turn plants—in this case, tobacco and lettuce—into little bioreactors by giving them “instructions” on how to make the desired proteins.
Shah’s work with mammalian cells is similar. Her group studies how flaviviruses like dengue and Zika virus hijack cellular processes to produce their own proteins—something they hope to imitate to increase biotechnology production. Both groups plan to make spike proteins using each type of cell and find out which host and production method produces the most proteins and the best results.
Faller’s group plans to help them both from the computational side. His group has is focused on finding out how different sugar distribution affects protein performance and thus has many existing models of glycosylated proteins, including models of COVID-19.
“If they are human-made or plant-made, they have different sugars around them, and as we make these proteins experimentally in different organisms, we need to understand how the different sugars impact the function of the protein,” he said.
A collaborative project
The goal is to create spike proteins that are not only fast and inexpensive to make, but also bind well to the antibodies. This is important to produce sensitive and accurate serological tests. In turn, Shah and McDonald’s experiments will help Faller’s group validate and refine their simulations, which will better inform the experiments, creating a beneficial feedback loop between the groups.
“This back-and-forth interaction is what makes this project possible,” said Faller. “As computational people, we need the experiments to validate our models. On the other hand, we have much better access to the direct molecular interactions than the experiments do.”
Though the CRAFT grant is short-term, the team hopes that this is just the beginning of their work toward fighting COVID-19. In the coming months, they plan to look into whether fragments of the protein could be made instead of the whole thing while producing the same results. Above all, they hope to develop effective biological production strategies for industry and future pandemics.
“These sorts of approaches would be applicable to many different scenarios where time is of the essence,” said Shah. “We’re applying it to COVID-19 here, but it’s not just a COVID-19 solution.”